Electrochemical CO2 reduction represents a promising way to make CO2 usable as a raw material for chemicals and fuels. In this article we look at the technical-economic aspects of CO2 electrolysis and highlight the profitability of some reaction products in different scenarios.
Evaluation criteria for CO2 electrolysis
Various criteria are used in the relevant literature to evaluate CO2 conversion technologies. Based on these indicators, we provide below an overview of the technical, energy-related and economic performance of electrochemical CO2 reduction (eCO2R):
- State of the art
- Energy performance
- CO2 usage
- Sustainability
- Capital expenditure (CAPEX)
- Operating expenditure (OPEX)
State of the art and energy performance
Electrochemical CO2 reduction is one of the simplest and most technically mature technologies for converting CO2 into useful products.
- Technological maturity (or the Technology Readiness Level – TRL) for C1 products is 4–8 (formic acid, synthesis gas); for C2+ products it is 1–3.2
- Electrochemical conversion systems can be specifically controlled via electrolysis parameters and are easy to handle and scalable.
- The environmentally friendly process uses water as the hydrogen source and can be carried out under mild conditions at close to room temperature and ambient pressure.
- The technology offers major advantages due to its high efficiency at moderate process temperatures and the possibility of using renewable energy sources directly for CO2 conversion.
CO2 usage and profitable products
CO2 can be converted via electrochemical reactions to simple compounds, for example formic acid, CO, methanol, propanol or even ethanol and ethylene. There is always great market potential for these CO2 electrolysis products, with formic acid (HCOOH) and its salts (formates), as well as carbon monoxide (CO), having emerged as the most economically viable products.
1. Market price
Based on normalised market prices (stored energy and market size), CO and formic acid are the most profitable products. Normalisation to the number of electrons needed to manufacture the products takes into account the electricity costs for manufacturing a product.
Although the market for these two products is limited, the possibility of turning CO and folic acid into more useful products makes them an attractive target product.
This assessment is based on various analyses, which we have already discussed in our article on the market potential of CO2-based products.3
2. Selectivity and process parameters
- A high degree of efficiency is desirable in order to minimise the separation processes required afterwards and thus keep capital and operating costs as low as possible.
- The faradaic efficiencies for CO and HCOOH are at least 80% at a current density of at least 300 mA/cm2.
- 2 moles of CO2 form 1 mole of formic acid and 1 mole of synthesis gas (mole = number of particles in a substance). Comparatively, formic acid and CO therefore have the best CO2 utilization values in terms of substance quantity.
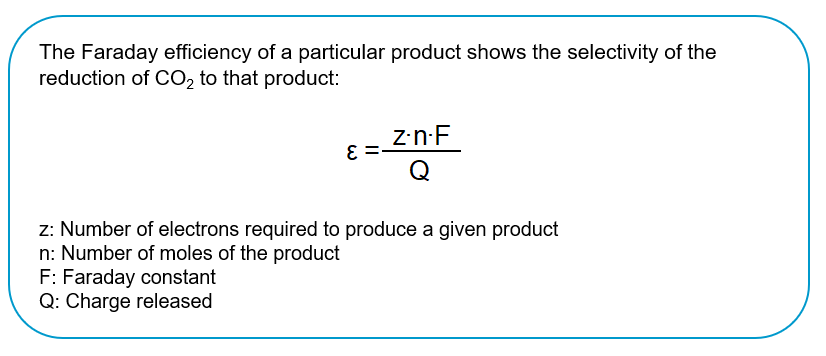
Figure 1: Faraday efficiency und selectivity of the reduction of CO2; in-house illustration
3. CO offers further advantages for CO2 electrolysis
Carbon monoxide is currently the most promising product for CO2 reduction, for the following reasons:
- CO is gaseous at ambient pressure.
- It is separable from aqueous electrolytes.
- It can be selectively produced with Ag catalysts.
- CO is an important chemical reactant that is widely used in today's chemical industry.
- CO can be converted into synthetic fuels and hydrocarbons using established Fischer-Tropsch processes.
CAPEX & OPEX: Detailed analysis for different CO2 products
In order to obtain a long-term overview, we have analysed various technical-economic results of CO2 electrolysis from the literature for different products and different process assumptions. Particularly meaningful are the results of the following analysis, which examines the process costs, including material consumption, capital and electricity costs, for various conversion products. 4
1. Process assumptions for the CO2 electrolysis model
Table 1: Process assumptions for a CO2 electrolyser model, adapted from 4
- Production rate: A rate of production of 100 tonnes per day equates to large-scale production. The capital costs are cheaper and the differences between the products are more recognisable, while the power requirement of the electrolysis system is at the scale of the largest commercial systems (∼100 MW).
- Service life and operating time: The service life of the electrolysis system was assumed to be 20 years, with two weeks off per year for maintenance work.
- Electricity price: An electricity price of $0.05 per kWh for the baseline scenario equates to the cheapest available electricity price at the time of the analysis (2018). Under the premise that renewable energy sources become cheaper, an electricity price of $0.03/kWh was chosen in the optimistic scenario.
- Power density / cell voltage: The electrolyser's total current density of 200 mA/cm2 has been demonstrated in numerous laboratory reactors at about 2.3 V. For the optimistic case, a current density of 300 mA/cm2 at a cell voltage of 2 V was assumed, which falls within the range of commercial water electrolysers.
- Product selectivity: Faradaic efficiencies of 90% have been demonstrated for numerous CO2 reduction products (e.g., CO, formic acid, methanol).
- Conversion: A baseline reactor conversion rate of 50% was chosen for the analysis, assuming that high selectivity can be achieved at this rate of conversion. An improved electrolyser design could potentially increase CO2 conversion to well over 50%.
- Net present value: In the financial model of the analysis, the net present value (NPV) of the system at the end of its life was calculated. It was assumed that the construction of the system would be completed in the first year and production would start in the second year. The working capital was assumed to be 5% of the capital investment, as well as an overall effective tax rate of 38.9%. Profit was calculated as the income from product sales minus the annual operating costs of the system.
2. Net present value
The net present value (NPV) analysis for the most important products shows that under the conditions of the baseline scenario, CO and formic acid would be the only profitable products for the CO2 electrolysis system. This is because CO and formic acid have the highest market value per electron. Improved catalytic performance is necessary for the profitability of alcohols.
Figure 2: End-of-life net present values of various chemicals produced by eCO2R under baseline and optimistic conditions. "X" means that the net present value has not been calculated because the annual net yield would be negative and thus production would be unprofitable. Adapted from 4
3. Investment and operating costs
In the detailed breakdown of process costs for each product, it is clear that formic acid and CO have the lowest electricity demand per kg of product, reducing the electricity costs and size of the electrolyser. In comparison, the low profitability of ethylene, for example, is caused by high electricity consumption and high demand for CO2 feedstock.
Figure 3: Investment and operating costs for the production of various chemicals under optimistic conditions. Adapted from 4
The Balance of Plant (BoP) costs were assumed to be 35% of the total costs of the electrolysis system. The BoP includes all supporting components and auxiliary systems of the CO2 electrolysis system.
It should also be noted that while distillation and PSA systems for separating liquid and gaseous products have similar investment requirements, pressure swing adsorption (PSA) has significantly lower operating costs. Although PSA is the cheaper process, the additional compression of the gas products required for transport and storage would probably drive up costs considerably.
4. Sensitivity analysis: Influence of parameters on the net present value
The sensitivity analysis illustrates the influence of different parameters on the profitability of the process. The range of values considered for each parameter is shown in the table below.
Table 2: Value range for the sensitivity analysis of the net present value. Adapted from 4
The most important results of the sensitivity analysis show the following picture:
- For all products, a 15% deviation in sales price had a significant impact on the NPV at the end of the service life. CO, n-propanol and formic acid, however, remained profitable despite falling sales prices.
- Furthermore, electricity is the most important operating cost for all products, with the exception of CO and formic acid, which means that there is a strong economic dependence on the price of electricity.
- For all other parameters, it can be seen that CO and formic acid have a significantly lower sensitivity compared to other products. Ethylene, for example, is strongly dependent on the current density: A reduction to 100 mA/cm2 would result in a $42 million reduction in NPV, while an increase to 500 mA/cm2 would only add an additional $8 million.
- From an economic-technical point of view, carbon monoxide and formic acid are the most promising candidates for large-scale chemical production.
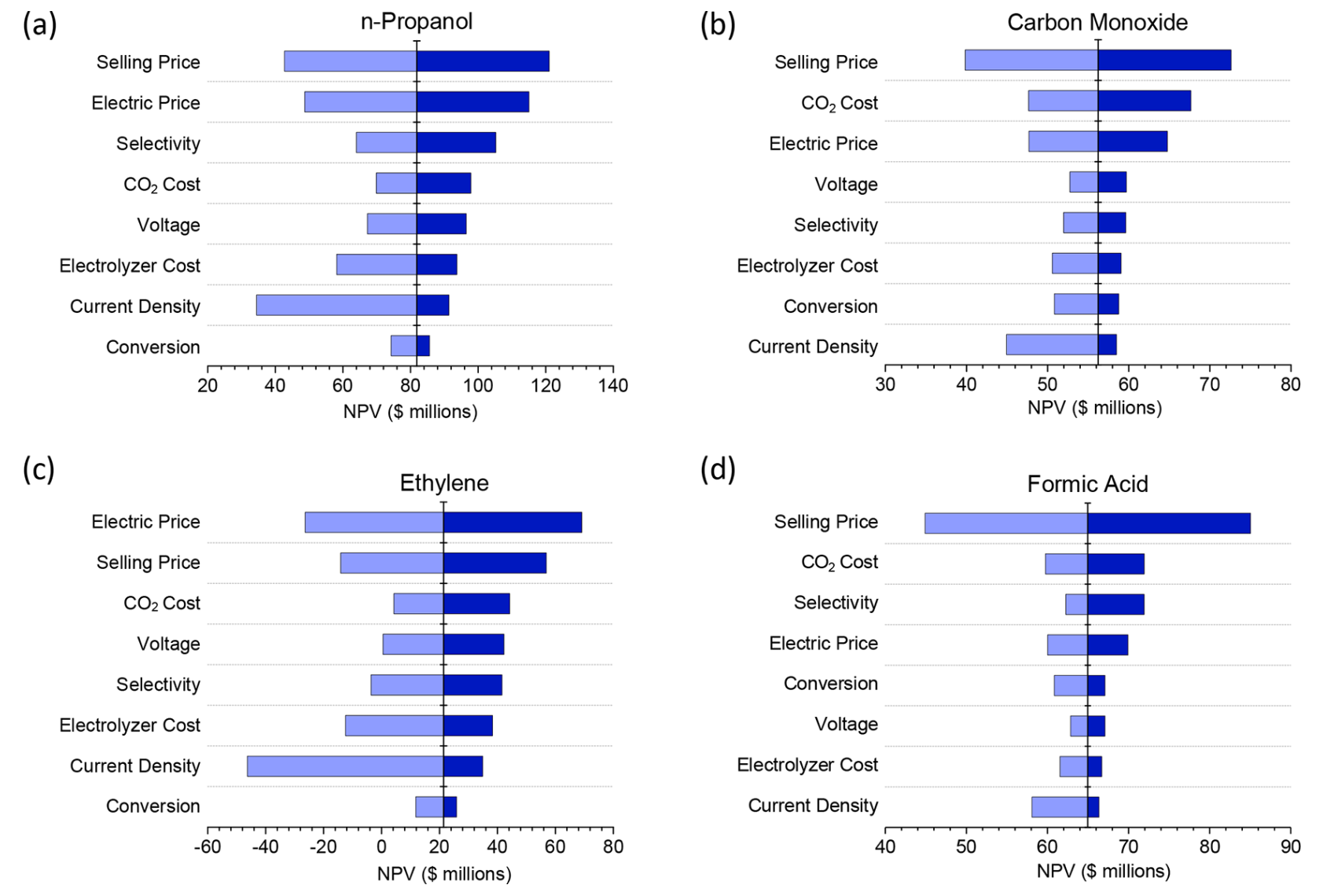
Figure 4: Sensitivity analysis of the net present value under optimistic conditions at the end of the service life for products from CO2 conversion (cf. Fig. 2). The centre line represents the net present value based on baseline sensitivity parameters. The dark blue bars show the effects of the parameters on the net present value in the "better" scenario; the light blue bars, the effects in the "worse" scenario (cf. Tab. 2). Adapted from 4
Where is the international trend heading?
Electrochemical CO2 reduction is an emerging technology that is highly likely to contribute to both energy storage and the supply of basic chemicals in the future.1 In particular, the reduction of CO2 to CO with subsequent processes to produce further value-added products, for example Fischer-Tropsch processes, can be a profitable avenue.
To bridge the "Valley of Death" and enable the commercial future of these processes, the focus of research must shift to materials that can be used on a large scale and are affordable. Close, interdisciplinary cooperation between science and industry is necessary to ensure technological progress in the field of CO2 electrolysis.
Product separation is also a complex and cost-intensive process, which is why it is desirable to obtain highly concentrated individual products. In the case of CO and formic acid, this criterion can already be met on a large scale.
GIG Karasek is looking for collaborators
Nobody can shape the future on their own. That is why GIG Karasek is looking for partners from research and industry to demonstrate the performance of CO2 conversion technology on a larger scale.
- With research cooperations and pilot systems, we want to accelerate technological progress and the market introduction of CO2 electrolysis.
- As a company active in plant and equipment engineering, GIG Karasek is able to "translate" research results and implement them on an industrial scale.
- As a specialist in the field of thermal separation technology, we have the necessary know-how to produce CO2 conversion products to a high quality and concentration.
We take over the entire project management, provide support in terms of operations and ensure the development of employees' skills. GIG Karasek thus offers added value over the entire service life of the system.
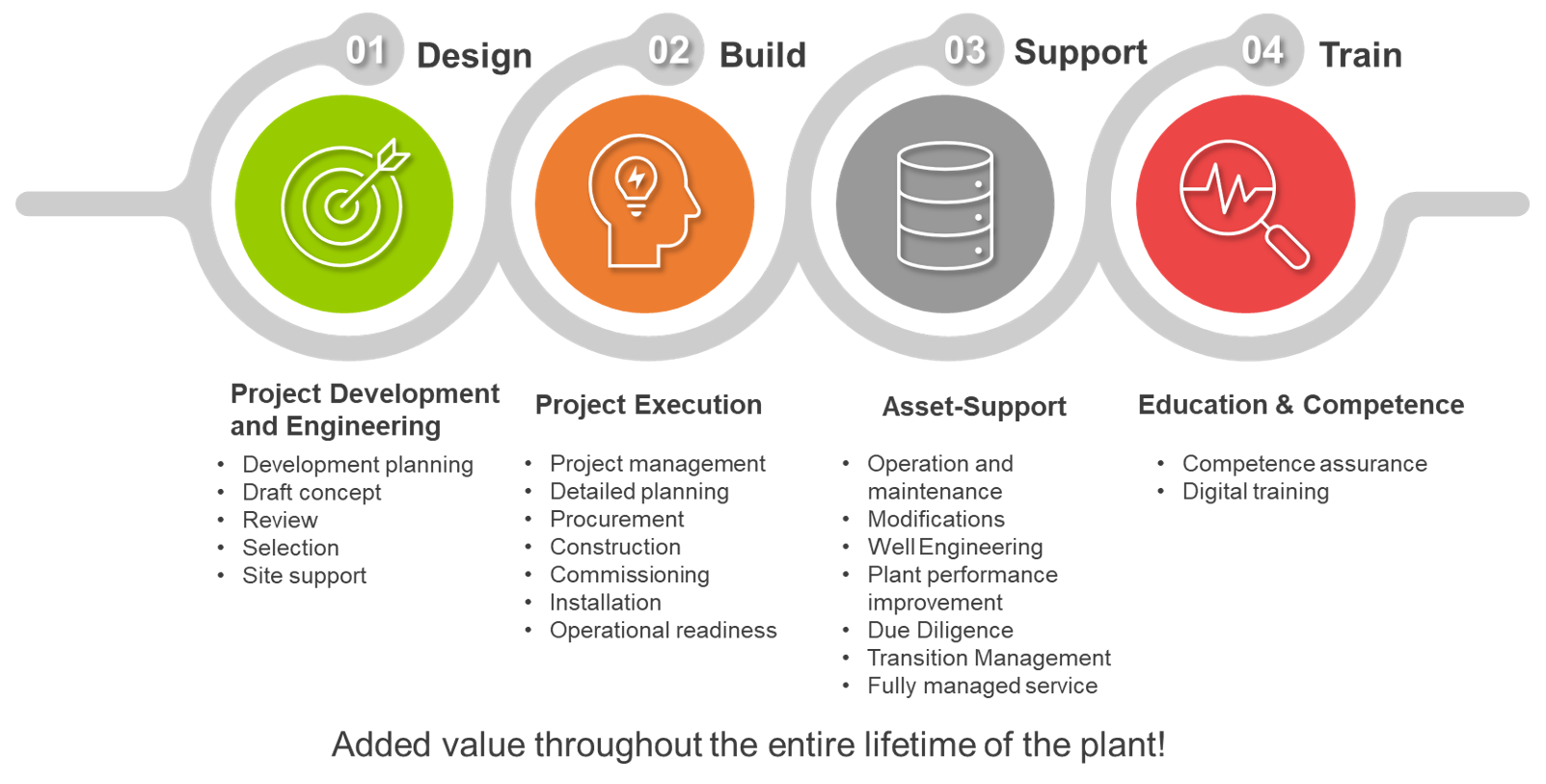
Figure 5: Example project sequence for research cooperations and pilot system projects; in-house illustration. © GIG Karasek
Conclusion: CO2 electrolysis
Simple products such as CO and formic acid are the most profitable under current economic conditions and based on the current state of the art in electrocatalysts. However, in order to bring the industrial application of CO2 electrolysis to market maturity, further research and pilot projects in a real industrial environment are required.
1 Mohammad Rezaei, M. B. A. (2023). CO2 utilization: new technologies for converting CO2 and why GIG Karasek opts for electrochemical reduction. Gigkarasek.com. Retrieved 22 February 2023, from https://www.gigkarasek.com/en/blog/co2-verwertung-methoden-herausforderungen
2 Nishikawa, E. (2022). CO2 conversion & utilization pathways: Techno-economic insights. PreScouter. Retrieved 22 February 2023, from https://www.prescouter.com/2022/04/co2-conversion-utilization-pathways/
3 Mohammad Rezaei, M. B. A. (2022). CO2-utilization: 5 reasons why companies should participate now. Gigkarasek.com. Retrieved 22 February 2023, from https://www.gigkarasek.com/en/blog/co2-verwertung-0
4 Jouny, M., Luc, W., & Jiao, F. (2018). General techno-economic analysis of CO2 electrolysis systems. Industrial & Engineering Chemistry Research, 57(6), 2165–2177. American Chemical Society. General Techno-Economic Analysis of CO2 Electrolysis Systems | Industrial & Engineering Chemistry Research (acs.org)